Journal Publications
View calorimetry lab chem101 (1).pdf from CHEM 101 at Pierce College. Objective: Part I: The purpose of part one was to determine the heat capacity of a calorimeter. Two Styrofoam cups were used. Welcome to the Chem 10X laboratories website. We are located on the second floor of the Gunning/Lemieux Chemistry Centre. On this website, you will find an array of useful information to help you make the most of your time in the laboratory classes. Read Book Chemistry 101 Laboratory Manual Pierce Chemistry 101 Laboratory Manual Pierce Texts: General, Organic and Biological Chemistry by Blei and Odian. Laboratory: Chemistry 121 Laboratory Manual Pierce College. Course Content: Chemistry 121 (formerly Chem 101) is the first quarter of a two-quarter Page 7/28. Golger, General Chemistry CH102 Laboratory Manual, (Hayden McNeil Publishing, 2018, ISBN 978-0-7380-8715-3). CH116 students do not need to purchase the CH102 Lab Manual and they will receive separate instructions about the lab. Laboratory notebook, Hayden McNeil Publishing; the 50-page version should be sufficient. As the CH 101 textbook, this lab course is not meant to follow the lecture course on a day-by-day basis. It is also not just a supplement to the lecture. Please consult your textbook and the lab website often for additional information on the topics covered in lab. Course Materials Lab Manual: Chemistry 102 Laboratory eManual.
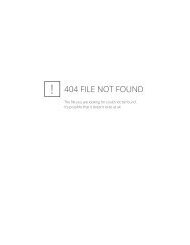
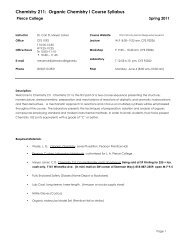
Citation data: Google Scholar
- A unified dynamic programming framework for the analysis of interacting nucleic acid strands: enhanced models, scalability, and speedACS Synth Biol, 9(10):2665-2678, 2020.
- Conditional guide RNAs: programmable conditional regulation of CRISPR/Cas function in bacterial and mammalian cells via dynamic RNA nanotechnologyACS Cent Sci, 5(7):1241-1249, 2019.
- Signal transduction in human cell lysate via dynamic RNA nanotechnologyACS Synth Biol, 7(12):2796-2802, 2018.
- Third-generation in situ hybridization chain reaction: multiplexed, quantitative, sensitive, versatile, robustDevelopment, 145:dev165753, 2018.
- Multidimensional quantitative analysis of mRNA expression within intact vertebrate embryosDevelopment, 145:dev156869, 2018.
- Constrained multistate sequence design for nucleic acid reaction pathway engineeringJ Am Chem Soc, 39:3134−3144, 2017.
- Mapping a multiplexed zoo of mRNA expressionDevelopment, 143:3632-3637, 2016.
- Single-molecule RNA detection at depth via hybridization chain reaction and tissue hydrogel embedding and clearingDevelopment, 143:2862-2867, 2016.
- Multiplexed miRNA northern blots via hybridization chain reactionNucleic Acids Res, 44(15):e129, 2016.
- Sequence design for a test tube of interacting nucleic acid strandsACS Synth Biol, 4(10):1086–1100, 2015.
- Combinatorial analysis of mRNA expression patterns in mouse embryos using hybridization chain reactionCold Spring Harb Protoc, 2015(3):259-268, 2015.
- Exquisite sequence selectivity with small conditional RNAsNano Lett, 14(8):4568-4572, 2014.
- Next-generation in situ hybridization chain reaction: higher gain, lower cost, greater durabilityACS Nano, 8(5):4284-4294, 2014. (open access)
- Developmental self-assembly of a DNA tetrahedronACS Nano, 8(4):3251-3259, 2014.
- Conditional Dicer substrate formation via shape and sequence transduction with small conditional RNAsJ Am Chem Soc, 135(46):17322-17330, 2013. (open access)
- Localizing transcripts to single cells suggests an important role of uncultured deltaproteobacteria in the termite gut hydrogen economy Proc Natl Acad Sci USA, 110(40):16163-16168, 2013.
- Selective nucleic acid capture with shielded covalent probes J Am Chem Soc, 135(26):9691-9699, 2013. (open access)
- Nucleic acid sequence design via efficient ensemble defect optimization J Comput Chem, 32:439-452, 2011.(pdf)(supp info)(supp structures)
- NUPACK: Analysis and design of nucleic acid systems J Comput Chem, 32:170-173, 2011.(pdf)
- Programmable in situ amplification for multiplexed imaging of mRNA expression Nature Biotechnol, 28:1208-1212, 2010.(pdf)(supp movie 1)(supp movie 2)(supp info)
- Selective cell death mediated by small conditional RNAs Proc Natl Acad Sci USA, 107(39):16777-16782, 2010.(pdf)(supp info)
Note: this paper has been retracted.Retraction for Venkataraman et al., Selective cell death mediated by small conditional RNAsProc Natl Acad Sci USA, 110(1):384, 2013. (pdf) - Programming biomolecular self-assembly pathways Nature, 451:318-322, 2008.
- An autonomous polymerization motor powered by DNA hybridizationNature Nanotech, 2(8):490-494, 2007.
- Thermodynamic analysis of interacting nucleic acid strandsSIAM Rev, 49(1):65-88, 2007.(pdf)
- Topological constraints in nucleic acid hybridization kineticsNucleic Acids Res, 33(13):4090-4095, 2005.(pdf)
- Triggered amplification by hybridization chain reactionProc Natl Acad Sci USA, 101(43):15275-15278, 2004.(pdf)
- A synthetic DNA walker for molecular transportJ Am Chem Soc, 126:10834-10835, 2004.
- Rewritable memory by controllable nanopatterning of DNANano Lett, 4(5):905-909, 2004.
- An algorithm for computing nucleic acid base-pairing probabilities including pseudoknots J Comput Chem, 25:1295-1304, 2004.(pdf)
- Adjoint and defect error bounding and correction for functional estimatesJ Comput Phys, 200:769-794, 2004.(pdf)
- Paradigms for computational nucleic acid designNucleic Acids Res, 32(4):1392-1403, 2004.
- A partition function algorithm for nucleic acid secondary structure including pseudoknotsJ Comput Chem, 24(13):1664-1677, 2003.
- Exact rotamer optimization for protein designJ Comput Chem, 24(2):232-243, 2003.(pdf)
- Algorithm developments for discrete adjoint methodsAIAA J, 41(2):198-205, 2003.(pdf)
- Protein design is NP-hardProtein Engineering, 15(10):779-782, 2002.(pdf)
- Analytic adjoint solutions for the quasi-one-dimensional Euler equationsJ Fluid Mech, 426:327-345, 2001.(pdf)
- Conformational splitting: a more powerful criterion for dead-end eliminationJ Comput Chem, 21(11):999-1009, 2000.(pdf)
- Adjoint recovery of superconvergent functionals from PDE approximationsSIAM Rev, 42(2):247-264, 2000.(pdf)
- An introduction to the adjoint approach to designFlow Turb Comb, 65:393-415, 2000.(pdf)
- Optimum aerodynamic design using the Navier-Stokes equationsTheor Comput Fluid Dyn, 10:213-237, 1998.(pdf)
- Efficient computation of unsteady viscous flows by an implicit preconditioned multigrid methodAIAA J, 36(3):401-408, 1998.(pdf)
- Preconditioned multigrid methods for compressible flow calculations on stretched meshesJ Comput Phys, 136:425-445, 1997.(pdf)
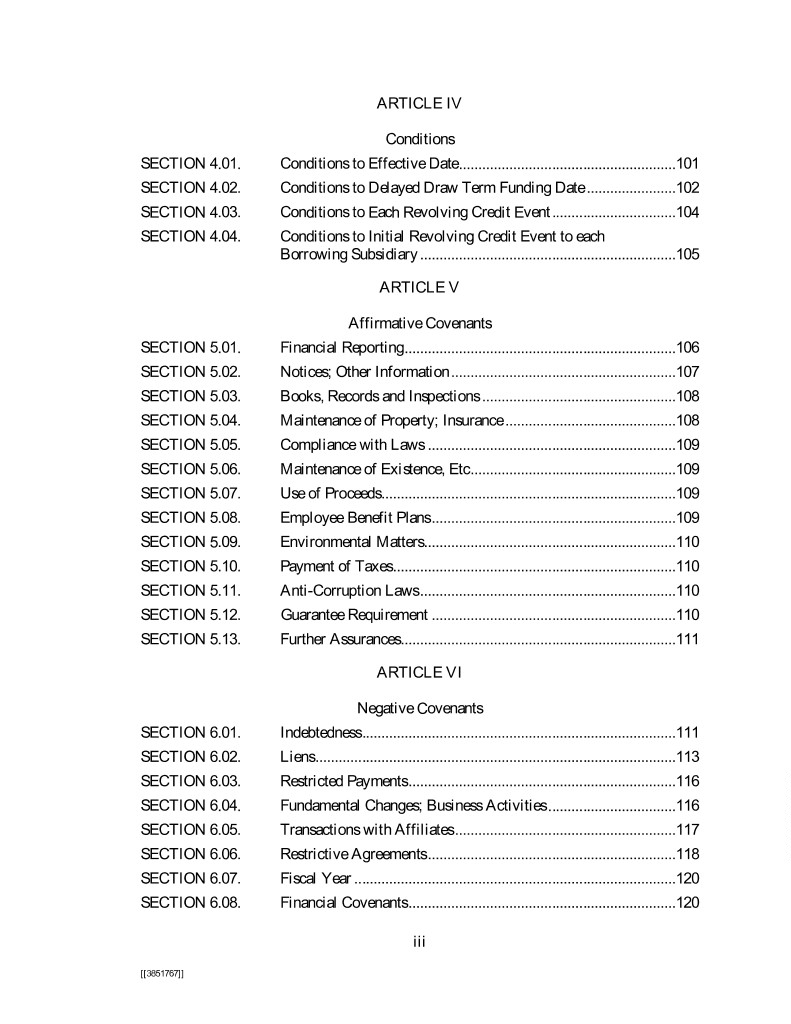
INTRODUCTION
Spectroscopy is the study of the interaction between matter and electromagnetic radiation. The types of electromagnetic radiation are often divided into the following broad categories (from short to long wavelengths): gamma radiation, X-ray radiation, ultraviolet radiation, visible radiation, infrared radiation, microwave radiation, and radio waves. Figure 1 illustrates the full electromagnetic spectrum.
Pierce Chem 101 Lab Manual Butkevich
Figure 1: The electromagnetic spectrum, with the visible spectrum highlighted
The visible spectrum of light is only one small sliver of the electromagnetic spectrum. It falls between approximately 400 nm to 700 nm, but our eyes distinguish the different wavelengths of light as different colors. The regions directly above and below this wavelength range are known as the infrared (IR) and ultraviolet (UV), respectively. The pigments in our eyes selectively absorb photons of the visible spectrum, but not those outside of it, because of their chemical makeup.
White light is a mixture of all visible wavelengths, which can be demonstrated by passing it through a prism or diffraction grating, which disperses the light into all of its component colors. When white light hits an object, some of the light may be reflected, some of it may be absorbed, and some of it may be transmitted (it passes through the object). Color arises from the selective absorption of photons of specific wavelengths. If the object is opaque, such as a wall, the reflected color is the complement of the color absorbed. If the object is transparent, such as a liquid, the transmitted color is the complement of the color absorbed. A spectrometer can separate the component colors coming either directly from an emission source or from the light transmitted through a sample. A top-down diagram of a spectrometer is shown in Figure 2.
Figure 2: The light path through a simple spectrometer
When the light from a simple emission source (like a single element) is viewed through a spectrometer, only specific lines of color are seen. Each of these lines corresponds to a unique electronic transition and the collection of lines is characteristic of the emission source. The emission spectrum for hydrogen is shown in Figure 3.

Figure 3: Production of the emission spectrum for hydrogen
Chem Lab Manual Pdf
A spectrophotometer is used to measure how much light is absorbed by a sample at varying wavelengths. In this instrument, a beam of monochromatic light is directed through a solution and the intensity of light transmitted (It) is compared to the intensity of the incident beam (Io), as shown in Figure 4. This fraction, sometimes expressed as a percentage, is termed the transmittance (T) of the sample at a particular wavelength:

[mathrm{T}=frac{mathrm{I}_{t}}{mathrm{I}_{o}}]
Figure 4: Simplified schematic of a spectrophotometer
Although it is sometimes useful to plot transmittance as a function of wavelength to get a transmittance spectrum, we will be focusing on absorbance (A), which is defined as:
[mathrm{A}=-log mathrm{T}=-log left(frac{I_{t}}{I_{o}}right)]
You can see from this equation that, as the transmittance diminishes, the absorption increases. Also, note that absorbance has no units.
In this experiment, you will use a spectrometer and a spectrophotometer to begin examining the different modes of light absorption and emission.
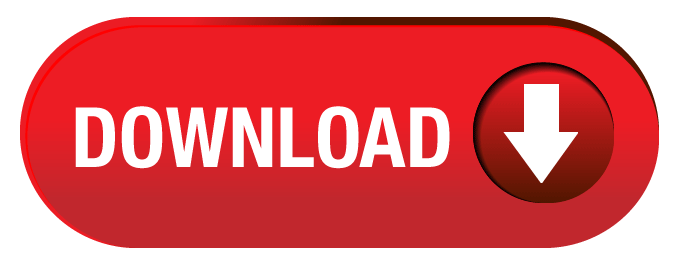